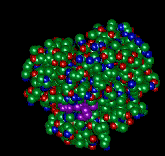
Myoglobin
stores oxygen in your muscles. The ligand binds to an iron atom
that is coordinated to a heme porphyrin (purple). The ligand is
also brightly colored in the above structure, but you can't see
it! How does it get out? |
Ultrafast
Protein Dynamics
Biological systems such as proteins, DNA, membranes,
cells, and organelles present spectacular challenges to our understanding
of chemical dynamics and structure. Most protein molecules have
reasonably well-defined structures to the extent that they can
be characterized by X-ray diffraction and NMR spectroscopy. These
structures, though, must necessarily respond to their environments
which can vary from solid to water to oily membranes (or all three).
Globular proteins, for example, are nearly solid density, and yet
to function they must often be flexible. Essentially, many biological
molecules are distinguished by the very fact that they are not
neatly categorized as solids or liquids, but are rather something
in between.
In order to push towards a detailed microscopic
description of these hard-to-classify biological systems, we are
developing an array of optical spectroscopy tools that will complement
the already commonly used X-ray and NMR techniques. We rely heavily
on state-of-the-art femtosecond (1 fs = 10-15 sec) laser pulses
and through various nonlinear optical processes we are able to
generate significantly intense pulses at any wavelength from the
ultraviolet to the infrared. Our main approach is to take advantage
of the rich chemical specificity and well-developed intuition of
vibrational transitions in order to track the course of chemical
events. Vibrational transitions can be excited both “resonantly” through
infrared absorption, or “nonresonantly” through Raman
scattering, and the information content relates directly to the
displacement of atoms, thus limiting our reliance on complicated
electronic transitions.
Our experimental approach is based on the workhorse
of multidimensional spectroscopy to find out how different motions
within a molecule, or between molecules are coupled together. NMR
has benefited from these advances for a number of years, and now
we are excited to be able to push them into the optical domain,
thus giving us access to unparalleled sub-picosecond temporal resolution.
These tools will offer us a new and exciting perspective on the
complex dynamics of biological molecules. |
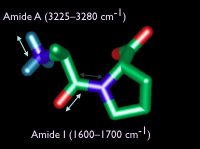
The amide bands of proteins are very sensitive
reporters of secondary structure. This proline-alanine didpeptide
shows the principal motions of the amide I (1600-1700 cm-1) and
the amide A (3225-3280 cm-1) bands. Most of the amide I mode is
carbonyl strech with a little carbon-nitrogen. The amide A is mostly
nitrogen-hydrogen motion. (C = green, N = blue, O = red, H = cyan) |